Current Projects
Several radioxenon isotopes (131mXe, 133mXe, 133Xe, 135Xe), each with a unique beta-gamma coincident decay signature, are characteristic byproducts of nuclear explosions. Due to the difficulty of containing noble gases, the detection of atmospheric radioxenon has been reliably utilized by the Comprehensive Nuclear Test Ban Treaty Organization (CTBTO) to confirm the nuclear nature of explosions. Each isotope can be separately identified by utilizing a coincident detection system.
The motivation for this project stems primarily from a backscatter effect observed in the TECZT system previously designed at Oregon State University (for more information on this project, see the TECZT tab). CZT is a dense and high-Z material, which make it excellent for detecting gamma radiation- however, these qualities also can cause electrons to scatter out of the crystal with only a partial energy deposition. In the TECZT system, beta particles and conversion electrons can deposit part of their energy in one CZT crystal, backscatter, and deposit the rest in the other crystal. This can be registered by the system as a coincident event, despite the fact that only one particle has been detected. Electrons might also deposit part of their energy in one CZT and scatter out of the system, while a coincident photon deposits its energy in the other CZT. This is a true coincidence event. Both of these situations are problematic: as radiation can come from a variety of sources, we need to be able to isolate a region of energies where we can say with a high degree of certainty that the energies deposited were from a coincident radioxenon decay (this is particularly important in the case of the conversion electron, which should be observed at a fixed energy, unlike betas which are emitted as a spectrum). If we account for these partial dispersion backscatters as possible coincident events, we run the risk of increasing the false positive rate; at the same time, if we simply disregard all the partial depositions to avoid these false positives, the overall efficiency of our system is reduced due to rejecting true coincidence events.
To address this problem, a prototype compact radioxenon detection system is being developed. The detector consists of a gas cell surrounded by a well type organic scintillator. This scintillator is used to detect beta radiation and conversion electrons. The base of the scintillator is coupled to an array of silicon photomultipliers for light readout, chosen for their low cost, low power demands, and ruggedness. A CZT crystal, chosen for its excellent energy resolution and room temperature operation, is coupled to the outer wall of the scintillator in order to detect coincident gamma radiation. The goal of this work is to produce a detection system with a minimum detectable concentration (MDC) for the radioxenon isotopes of interest comparable to other state of the art radioxenon detection systems, such as the Swedish Automatic Unit for Noble gas Acquisition (SAUNA) and the Automated Radioxenon Sampler and Analyzer (ARSA), at a fraction of the cost.
The Radiation Compass is a direction-sensitive radiation detector designed for use as part of a low-cost, autonomous radiological search tool based on an unmanned aerial system (UAS) platform. The normalized count rate from the symmetric panel array forms a characteristic detector response, generated by the passive masking of the detector panels, that is used to estimate the direction in which the radiological source is located. The Radiation Compass was designed and built using low-cost, lightweight, rugged components to afford the UAS-based system wide applicability and long flight times. The UAS-based radiological search system would remove the need for human teams to search potentially hazardous areas in emergency response, national security, and battlefield assessment scenarios
Intensity of detector panel response to incident radiation
The radiation compass attached to a drone
A live demonstration of the directional capabilities of the radiation compass
The OSU Compact Gamma Spectrometer (OCGS) was designed as a compact, low-cost gamma-ray spectrometer that can easily be wirelessly connected to a personal mobile device for on-the-go radioisotope measurement, identification, and personal dosimetry. The OCGS is based on a silicon photomultiplier (SiPM) and FPGA-based digital pulsed processor. The SiPM has several advantages compared to the more traditional PMT, including better durability, insensitivity to magnetic fields, smaller size, and lower bias voltage. A prototype device using a CsI(Tl) scintillator crystal has been assembled and tested in our laboratory at OSU. The OCGS is also easily able to be adapted to neutron detection by using different scintillator materials, such as CLLB and Stilbene.
Temporal gamma-ray spectroscopy is being used at OSU to estimate the amount of fissile material in a sample. A fast-rabbit sample transfer system was set up in the Oregon State University TRIGA Reactor (ORST) to irradiate samples and then quickly move them to the counting position in front of a high-purity germanium detector. This non-destructive assay method uses the time-dependent characteristics of gamma-ray peaks from fission product decay and in-growth instead of nuclear cross-section data, which can carry significant uncertainty. The method initially developed and demonstrated at OSU using a list-mode readout is being developed for use in real-time using a high-performance FPGA.
An eight-channel, high-performance digital pulse processor, the DPP3, is being developed for use with both multi-detector radioxenon detection systems and with the temporal gamma-ray spectroscopy system. The DPP3 has two parts: an analog front-end PCB and the FPGA module. Each channel on the analog front-end PCB has an adjustable voltage offset, and a Nqyuist filter leading to a 14-bit, 125 MHz 8-channel ADC, which has a 2-lane LVDS serial output. The ADC outputs, as well as a 24-pin GPIO header, are connected to the FPGA module via FPGA mezzanine connector (FMC). The FPGA module hosts a Xilinx Kintex-7 high-performance FPGA and a high-speed USB 3.0 interface to a PC. Pulse processing for all eight channels will take place in the FPGA, allowing full control over processing techniques and implementation.
Detection of xenon isotopes (radioxenon) has proven to be an important method for detecting nuclear explosions and is particularly well suited to detecting undeclared underground testing. The radioxenon isotopes 131mXe (t1/2 = 11.934 d), 133mXe (t1/2 = 2.19 d), 133Xe (t1/2 = 5.243 d) and 135Xe (t1/2 = 9.14 h) are produced in significant amounts in nuclear explosions and are of particularly high-value in identifying such events and thus are the focus of current radioxenon detection systems. The sensitivity of these devices which is determined as the Minimum Detectable Concentration (MDC) must be high enough to detect an ultra-low concentration of radioxenon in the atmosphere. International Monitoring System (IMS) which is a worldwide network of observational technology helping to detect and confirm violations of the Comprehensive Nuclear Test-Ban Treaty (CTBT) requires that the MDC of all radioxenon detection system be less than or equal to 1 mBq/m3 for 133Xe.
The main objective of this research is to design and develop a new radioxenon detection system which is small and compact with a minimal number of channels which reduces complexity, power and volume and still very good energy resolution can be achieved. Improvements of the available radioxenon detection system used to detect radioactive xenon in the atmosphere in order to discover clandestine nuclear test explosions were the main focus of this research.
This radioxenon detection system was designed and developed based on room temperature semiconductor materials at the Radiation Detection and Dosimetry lab at Oregon State University. The system uses two face-to-face coplanar CdZnTe (CZT) crystals for coincidence detection of beta/conversion electrons and gamma/x-rays. A two channel pulse processor with Field Programmable Gate Array (FPGA) processes anode pulses from the detector.
The detection system was characterized for radioactive lab sources and four radioxenons of interest and showed promising results. The MDC of our detection system for 133Xe is below 1 mBq/m3 which is compatible with IMS requirements.
Proximity charge sensing is a relatively new technique for semiconductor radiation detectors that has a few distinct advantages over directly-deposited electrodes. The first advantage is that it eliminates the need to deposit electrodes on the semiconductor crystal, which can simplify the fabrication process and reduces the over all cost of the detector. Second, proximity charge sensing reduces the leakage current associated with directly depositing the electrodes on the semiconductor surface. Finally, in position sensitive systems, it can be used to improve the position sensitivity of the device via signal interpolation. The advantages of using proximity electrodes have been previously demonstrated by their implementation in Ge and Si-based detectors. Though the energy resolution of HPGe detectors has not yet been surpassed, room-temperature semiconductors such as CZT offer a significant advantage over HPGe since they do not require expensive, high-maintenance cooling systems.
The research being done at Oregon State University explores the use of proximity charge-sensing on CZT crystals. It includes simulations to calculate the weighting potentials and electric fields for different coplanar electrode designs and fabricate proximity electrodes on compound semiconductors, such as: (1) a high resistivity contact to be applied on the proximity surface (anode side) that should not affect the induced charge generated from radiation interaction and should not trap charges at the detector surface, (2) a proper metal to serve as an Ohmic contact to dissipate charges accumulated on the crystal surface, (3) dielectric material to isolate the detector from the electrodes, and (4) proximity-electrodes that are implemented in this research on a PCB (Rogers 4350). The weighting potentials of proximity-sensing electrodes and directly-deposited electrodes are generated using ANSYS Maxwell and quantitatively compared using a Figure of Merit (FOM), which compares the designs in terms of uniformity and similarity of the generated weighting potential. The most promising proximity-sensing electrode design is currently being fabricated and characterized using a (19.4 mm x 19.4 mm x 5 mm) CZT crystal, and its performance is being compared to directly-deposited electrodes.
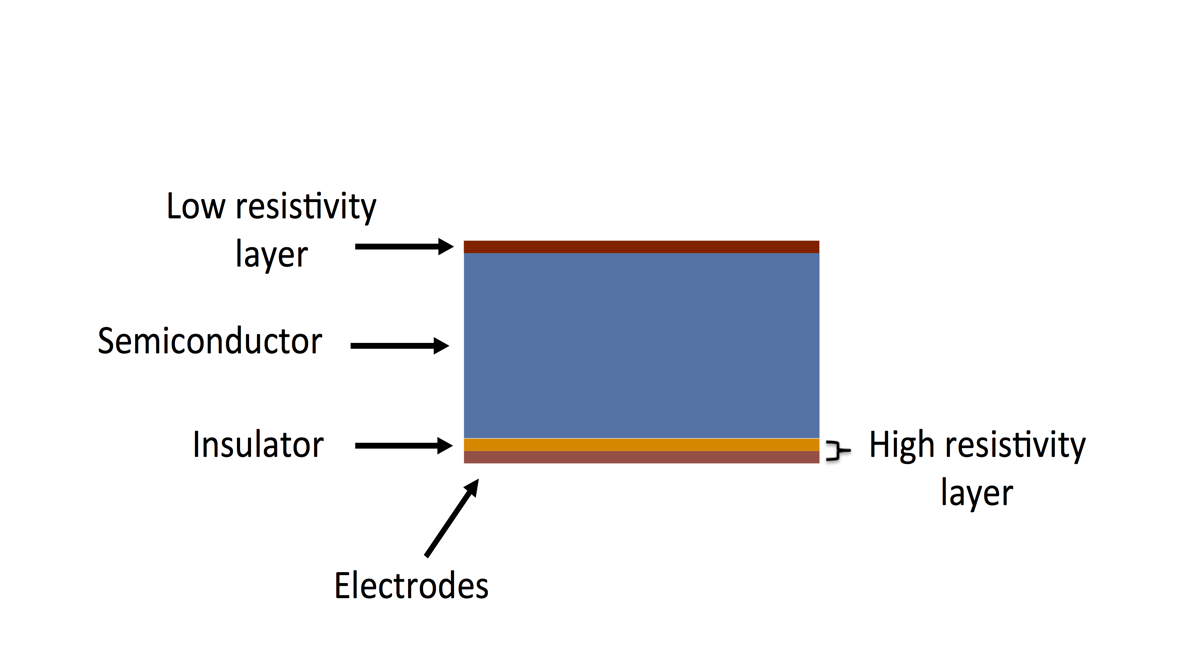
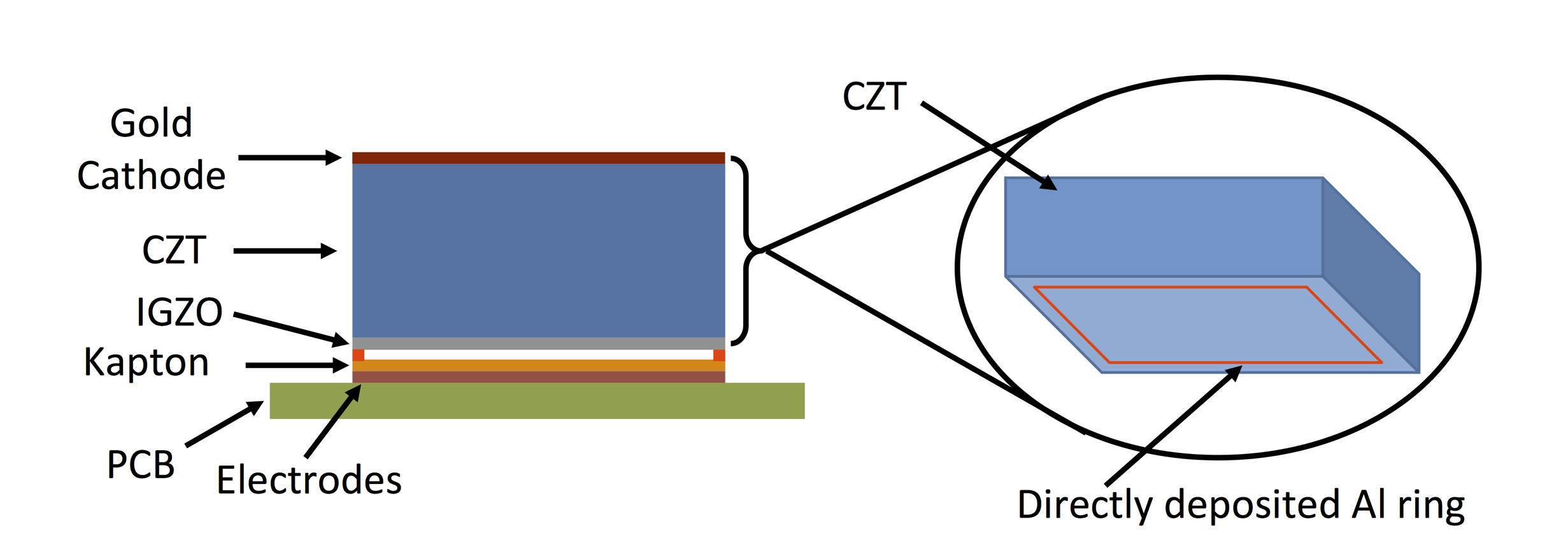